Space exploration increasingly relies on small satellites, particularly nanosatellites like CubeSats, to carry out various missions. As the number of CubeSats launched continues to grow, the demand for technologies that enhance their functionality also rises.
One such innovation is the electro-mechanical docking mechanism designed specifically for CubeSats, which is the focus of the upcoming DockSat mission. This article, based on the research conducted by Gideon Serfontein under the supervision of Drs. Lourens Visagie and Willem Jordaan, explore the development, testing, and significance of this docking system.
The Need for CubeSat Docking
Nanosatellites, typically weighing between 1 kg and 10 kg, have gained substantial traction in recent years. In 2021, approximately 326 nanosatellites were launched, and projections indicate that this number could exceed 2,080 by 2028. CubeSats, a popular type of nanosatellite standardised in 10 cm cubes, have made space more accessible to universities, startups, and research institutions by offering a cost-effective platform for various missions.
While docking technology is well-established for larger spacecraft like the Space Shuttle and the International Space Station, it remains largely unexplored for CubeSats. Docking small satellites presents unique challenges due to their limited size and resources. Precision and reliability are critical, and traditional propulsion methods are often unsuitable. This project aims to address these challenges by developing an electro-mechanical docking mechanism designed for CubeSats.
Docking mechanisms enable spacecraft to connect and work together in space. For larger satellites, this technology is mature and routinely used. However, for CubeSats, docking is a relatively new concept. The small size of CubeSats means that traditional docking systems are too bulky and consume too much power. Additionally, maintaining accurate control over the satellites’ movements is more challenging at this scale.
Electromagnets offer a promising alternative to fuel-based propulsion for manoeuvring CubeSats. They provide precise control without the need for propellant, which is advantageous given the limited resources on small satellites. Integrating electromagnets with a docking mechanism makes it possible to achieve the accuracy required for successful docking operations.
Designing the Docking System
This project builds upon the foundational work of Robert Waller, who designed an initial docking mechanism and explored using LED fiducial markers for pose estimation—the process of determining the satellites’ positions and orientations relative to each other. The goal was to enhance and expand this system to make it more reliable and suitable for real-world applications.
Key Components
- Docking Adapter and Locking Mechanism: The docking adapter is the component that physically connects two CubeSats. It was redesigned to be stronger and easier to manufacture. The locking mechanism ensures that once the satellites are connected, they remain securely docked, even if there are slight misalignments during the approach.
- Pose Estimation System: Accurate pose estimation is crucial for successful docking. This system uses LED markers and a camera to determine the exact positions and orientations of the satellites. This information feeds into a control system that guides the satellites into the correct docking position.
- Electromagnetic Coils and Control Algorithms: Instead of relying on traditional propulsion, electromagnetic coils are used to control the satellites’ movements. These coils generate magnetic fields that adjust the satellites’ positions and orientations. PID (Proportional-Integral-Derivative) controllers were developed to manage these adjustments, ensuring smooth and precise docking manoeuvres.
- Printed Circuit Board (PCB) Integration: A custom PCB was designed to integrate all electronic components, including the electromagnetic coils and LED markers. This compact design ensures that everything fits neatly within the small form factor of a CubeSat.
Testing and Verification
Each part of the docking system underwent thorough testing to ensure it meets the necessary standards for reliability and performance.
Docking Adapter Testing
The redesigned docking adapter was tested to confirm it could handle the required forces. Specialised rigs were used to assess the adapter’s ability to maintain precision even when there were slight misalignments. These tests demonstrated that the adapter could reliably secure two CubeSats together under various conditions.
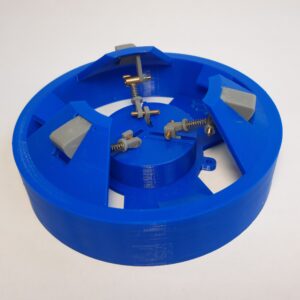
Fig 1: Prototype of the initial docking adapter
Pose Estimation Testing
To verify the accuracy of the pose estimation system, controlled experiments were conducted where the target satellite was positioned at precise offsets. By capturing images and analysing the LED markers, the system successfully determined the satellites’ relative positions and orientations. These tests confirmed that the pose estimation system is accurate enough for reliable docking.
Electromagnetic Coil Testing
Using Finite Element Analysis (FEA) software, the performance of the electromagnetic coils was simulated. Prototype tests measured the actual magnetic field strengths, confirming that the coils could generate the necessary forces to control the satellites’ movements effectively.
Control System Testing
Simulations in both two and three dimensions were conducted to evaluate the control algorithms. The PID controllers effectively managed the electromagnets and reaction wheels, guiding the satellites from a standstill to a successful dock in the simulations. These results indicate that the control scheme is reliable for real-world applications.
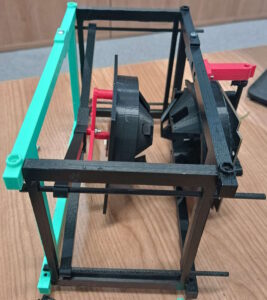
Fig 2: Frame to hold two docking adapters at certain misalignments and test if docking is successful.
Outcomes
The project successfully met all the objectives outlined at the beginning with the following key outcomes:
The redesigned docking adapter performed exceptionally well under the specified force conditions, as confirmed through extensive mass testing. Additionally, precision docking was achieved within the predefined misalignment ranges using specialized rigs. This ensured that the CubeSats could securely connect even when slight misalignments occurred during the docking process, highlighting the adapter’s robustness and reliability.
The pose estimation system proved to be highly effective in determining the satellites’ positions accurately from captured images. Through controlled experiments, the system successfully measured the target satellite’s orientation by altering its pitch and analyzing the resulting data. These precise orientation measurements were crucial in ensuring that docking manoeuvres were reliable and could be executed smoothly, thereby enhancing the overall accuracy of the docking process.
The performance of the electromagnetic coils was thoroughly validated through both simulations using Finite Element Analysis (FEA) software and prototype testing with a magnetometer. These tests confirmed that the coils could generate the necessary magnetic fields to control the satellites’ movements effectively. Furthermore, the developed PID control algorithms successfully guided the satellites from a standstill to a docked state during simulations. This demonstrated that the control scheme was both effective and reliable, ensuring smooth and precise docking manoeuvres.
These results confirm that the docking system is ready for the DockSat mission, paving the way for successful autonomous CubeSat docking.
Future Work and Recommendations
While the docking system is prepared for the DockSat mission, further improvements and testing are necessary to ensure its readiness for space deployment:
- Material Advancement: Transitioning from prototype materials to aerospace-grade materials will enhance the system’s durability and reliability in space conditions.
- Integration with CubeSat Systems: Ensuring compatibility with various CubeSat architectures, power systems, and on-board computers will make the docking system more versatile.
- Launch Preparation Tests: Simulating launch conditions through vibration testing will verify that the docking mechanism can withstand the stresses of launch without compromising its functionality.
- Docking Maneuver Simulations: Practical tests using an air-bearing trolley system will replicate the frictionless environment of space, allowing validation of the control scheme and mechanical reliability of the docking adapter.
- Control Scheme Optimization: Enhancing the control algorithms will improve their reliability and adaptability, enabling the system to handle a broader range of scenarios.
- Wireless Communication Integration: Developing wireless protocols will facilitate seamless data transfer and command exchange between docked CubeSats, enhancing their cooperative mission capabilities.
- Extended Duration Testing: Long-term testing of docking and undocking cycles will assess the system’s durability and ensure it can handle the expected number of operations over the CubeSats’ lifespans.
- Thermal Vacuum Testing: Simulating the extreme temperatures and vacuum conditions of space will ensure that the docking system’s materials and electronics remain functional without degradation.
These steps will refine the docking system, making it more reliable and versatile for various CubeSat missions.
Final Thoughts
Developing an electro-mechanical docking mechanism for CubeSats represents a significant advancement in small satellite technology. By addressing the challenges of docking at a small scale—such as precise control and limited resources—the project has created a system ready for real-world application in the DockSat mission.
This innovation opens up new possibilities for CubeSat operations, including satellite servicing, in-space assembly, and collaborative missions that were previously only feasible with larger spacecraft. As space exploration continues to evolve, advancements like this docking mechanism will play a crucial role in shaping the future of satellite missions.
Read the full research paper at https://scholar.sun.ac.za/items/0761241a-0a0a-4a2a-8ff2-3cf7f87cd5e2